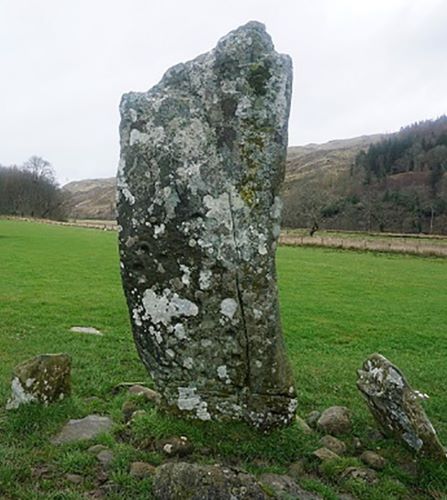
Examining the material effects of climate change on cultural heritage.
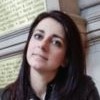
By Dr. Elena Sesana
Professor of Architecture and Engineering
Norwegian University of Technology and Science
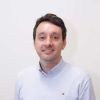
By Dr. Alexandre Gagnon
Senior Lecturer
School of Biological and Environmental Sciences
Liverpool John Moores University
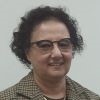
By Dr. JoAnn Cassar
Professor of Conservation and Built Heritage
L-Università ta’ Malta
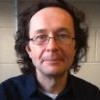
By Dr. John J. Hughes
Reader
School of Computing, Engineering and Physical Sciences
University of the West of Scotland
Abstract
Climate change, as revealed by gradual changes in temperature, precipitation, atmospheric moisture, and wind intensity, as well as sea level rise and changes in the occurrence of extreme events, is already affecting cultural heritage sites. Accordingly, there is a rapidly increasing body of research reporting on the impacts of climatic stressors on cultural heritage and on the assessment of climate change impacts on cultural heritage assets. This review synthesizes the international literature on climate change impacts on tangible cultural heritage by developing hazard-impact diagrams focusing on the impacts of gradual changes in climate on: (1) the cultural heritage exposed to the outside environment, (2) the interiors of historical buildings and their collections, and (3) a third diagram associated with climate change and the impacts due to sudden changes in the natural physical environment (e.g., storm surges, floods and landslides, wildfire) in addition to sea level rise, permafrost thawing, desertification and changes in the properties of the oceans. These diagrams, which depict the relationships between various stressors and their impacts on cultural heritage, will allow other researchers, stakeholders, and potentially decision makers to determine the potential impacts of climate change on a specific cultural heritage asset without a separate examination of the literature. This review thus provides the current state-of-the-art on the impacts of climate change on the tangible, built heritage, that is, monuments, archeological sites, historical buildings, as well as their interiors and the collections they hold, highlights the limitations of previous research, and provides recommendations for further studies.
Introduction
Cultural heritage assets such as historical buildings, archeological sites, and monuments, their contents and collections, as well as their intangible aspects are a legacy from our past that lend a sense of place, identity, and aesthetic wellbeing to local populations (M. Cassar, 2009; Phillips, 2015). These heritage assets have always been and will continue to be subjected to interactions with their environment, often referred to as weathering processes, and thus to change. Climate change is an additional potential threat as it exacerbates the expected rates of decay and/or contributes to the appearance of new decay phenomena (Bertolin, 2019). This is because climatic changes may aggravate the physical, chemical, and biological mechanisms causing degradation by affecting the structure and/or composition of the affected materials. Climate change may also influence the frequency and intensity of hazardous events such as droughts, floods and landslides with inevitable widespread impacts, also on cultural heritage. Moreover, cultural heritage is at risk from Sea Level Rise (SLR), changes in the intensity of storm surges, with associated impacts on coastal erosion, flooding and potentially inundation, in addition to changes to inland fluvial dynamics (Howard et al., 2008; ICOMOS Climate Change and Heritage Working Group, 2019; Sabbioni et al., 2010; UNESCO World Heritage Centre, 2007, 2008). For these reasons, there has been a growing body of research investigating the impacts of these climatic stressors and other changes in the natural physical environment on heritage typologies and materials.
Changes in temperature, precipitation, and atmospheric moisture, and wind intensity, in addition to SLR, desertification, and the interaction between climatic changes and air pollution have been identified by the United Nations Educational, Scientific and Cultural Organization (UNESCO) as threats to cultural heritage (UNESCO World Heritage Centre, 2007), leading to the issuing of a policy document the following year (UNESCO World Heritage Centre, 2008), stimulating a growing body of related research. More recently, a report published by the International Council on Monuments and Sites (ICOMOS; ICOMOS Climate Change and Heritage Working Group, 2019) summarized the key climatic factors and their mechanisms and impacts derived from consultations with experts. This is also one of the aims of the current review, but it synthesizes predictions of change deriving from previous scientific literature rather than from expert knowledge. Although Fatorić and Seekamp (2017) have previously reviewed the literature on climate change and cultural heritage, they focused on trends in research, including geographical scope, methodological approach, and type of cultural heritage investigated, rather than synthesizing the state of knowledge on the impacts of climatic stressors on heritage assets. The latter was done in Daly (2013), albeit limiting the review to archeological sites. The current article, on the other hand, is not limited to one heritage typology and is global, encompassing examples from different climate zones and also examines the interactions and complexity of multiple climatic stressors on cultural heritage, organized and presented across three themed sections. The first and second sections examine the impacts of gradual changes in climate on the heritage exposed to the outdoor environment, and on the indoor heritage with its collections, respectively. The third section investigates the risks from sudden changes in the natural physical environment on cultural heritage, such as storm surges, floods, and landslides. Considering the Fifth Assessment Report (AR5) Intergovernmental Panel on Climate Change (IPCC, 2014) risk terminology, this review focuses on the material effects as a result of changes in the hazard; it does not consider the vulnerability of the cultural heritage asset.
Methods
A systematic literature search was conducted in English using ScienceDirect and Google Scholar. The details of the Boolean search strategy are provided as Supporting Information; it began with the keywords “climate change” AND “cultural heritage,” which resulted in 3348 articles using ScienceDirect, for instance. Using these two keywords led to numerous articles on a variety of related topics, including adaptation, mitigation, disaster risk reduction, urbanization, tourism, to name a few. The authors went through the first 200 articles resulting from the search and selected the relevant outputs. The literature search was then narrowed down by adding further keywords. Accordingly, the term “impact” was added, but the addition of this third keyword only decreased the number of articles to 2988. The review of the literature continued by adding other keywords, related to the known mechanisms causing degradation of cultural heritage and the specific impacts of climate change. These included, for instance, “freezing and thawing,” “thermoclastism,” “corrosion,” and “biological degradation.” The literature search using Google Scholar identified key reports funded by the European Commission (e.g., NOAH’s ARK,1 Climate for Culture2 [CfC]), as well as those by UNESCO and national heritage organizations. The publication lists of the authors directly and indirectly associated with these reports, as identified from the systematic literature review already carried out, were consulted as well as relevant websites, to validate the search ensuring its robustness.
The authors considered 100 outputs (journal articles, book chapters, and peer-reviewed conference proceedings), published from 1999 to July 2020, as well as reports from international institutions (Table 1). Additional publications appraised included review articles and project reports, as well as other original research articles, with a total of 191 references cited in this review. It was found that the issue of climate change and its impacts on cultural heritage were first mentioned in the 1990s in Australia (Rowland, 1992, 1999); with a hiatus in publications until the NOAH’s ARK project during 2004–2007 (Sabbioni et al., 2006). One exception was the article by Viles (2002), on climate change and stone degradation, followed by more broad-based opinion article on climate change and heritage impacts by Brimblecombe (2003). Region-specific studies (e.g., one based in the UK) then also followed with a publication by M. Cassar and Pender (2005). However, it was only in the AR5 report, that is, IPCC (2014), that the IPCC first mentioned the impacts of climate change on cultural heritage (Sesana et al., 2018).
The publications identified were divided into three groups based on their content: (i) impacts of gradual changes in climate accentuating the typically slow rate of material degradation of outdoor heritage; (ii) impacts of gradual changes in climate on the indoor heritage and collections, which, as in (i), can enhance the rate of degradation processes; and (iii) impacts of sudden changes in climatic stressors, for instance extreme precipitation leading to floods, causing abrupt damage, or leading to environmental conditions enhancing the rate of material degradation (Table 1). Sub-categories highlighted specific decay mechanisms affected by the change in climate on the outdoor and indoor cultural heritage for Sections 3 and 4, respectively, and in categories related to changes in the natural physical environment for Section 5.
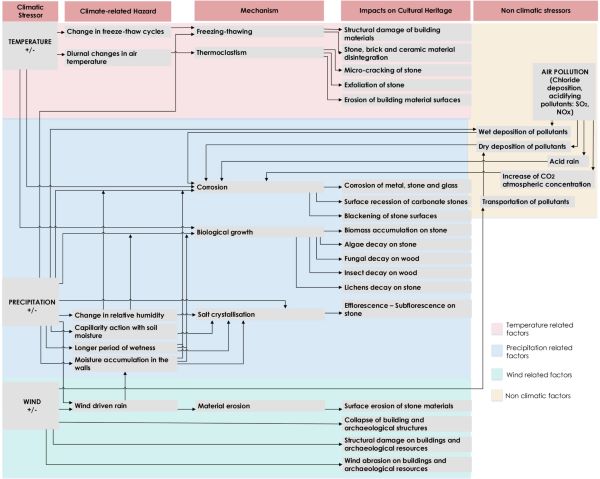
Three impact diagrams were then developed to synthesize the relationships between climatic stressors and changes in the rate of decay of cultural heritage materials and structures, as reported in the literature. These diagrams also depict the impacts of multiple synergistic climatic stressors and their interconnections. The first diagram shows the impacts of gradual variations in temperature, precipitation and wind on outdoor heritage and thus directly exposed (Figure 1).
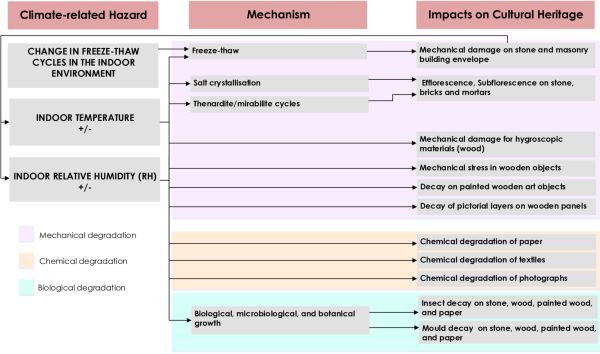
The second diagram summarizes the impacts of gradual variations in temperature and humidity on the indoor cultural heritage, including collections (Figure 2).
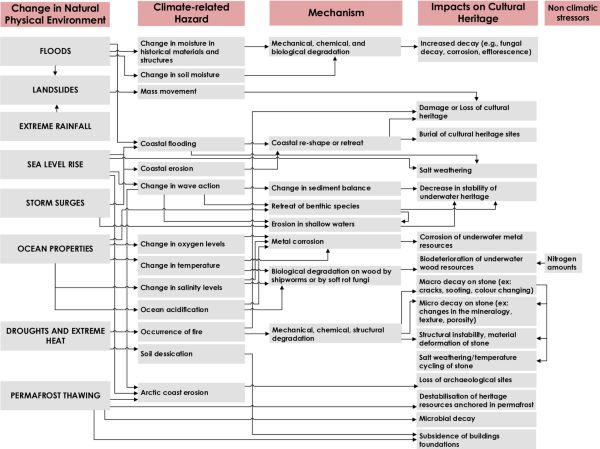
The third diagram presents the state of knowledge on the impacts of changes in the natural physical environment on heritage, such as fluvial floods and more gradual changes in fluvial dynamics; SLR, storm surges and their coastal impacts; landslides; extreme heat; and changes in the properties of the ocean (Figure 3).
Impacts of Gradual Changes in Climate on the Outdoor Cultural Heritage
Overview
This section reviews the literature investigating the impacts of gradual changes in temperature, precipitation, humidity, and wind on the mechanisms causing degradation of heritage exposed to the outdoor environment.
Changes in Temperature
Freeze-Thaw Cycles
During freezing, water held in porous materials increases in volume, causing internal stress (Sabbioni et al., 2010). Although the freezing–thawing mechanism is projected to occur less frequently under climate change for most of Europe (Brimblecombe & Grossi, 2007; Brimblecombe, Grossi, & Harris, 2011; Camuffo, 2019; Grossi et al., 2007; Nijland et al., 2009; Sabbioni et al., 2010; Viles, 2002), it could increase in some regions. In the far North of Europe, a warmer climate would allow temperatures to oscillate above and below the freezing point, endangering porous materials in archeological sites and historical buildings (Grossi et al., 2007; Haugen & Mattsson, 2011; Sabbioni et al., 2008; Sabbioni et al., 2010). The resulting mechanical weathering can lead to structural damage and disintegration of stone, brick, and ceramic materials (Figure 1; Grossi et al., 2007; Sabbioni et al., 2008).
Thermoclastism
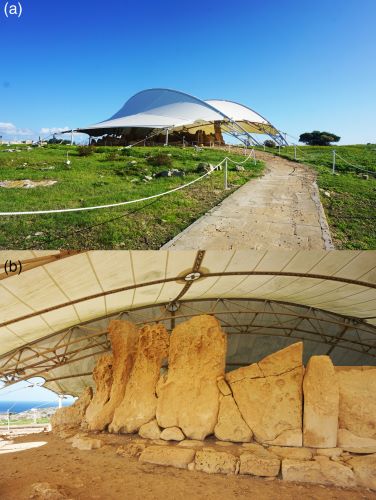
Thermoclastism results from expansion and contraction of surface mineral grains due to thermal variations caused by seasonal variations and diurnal changes in air temperature and direct insolation (Yaldiz, 2010), and can lead to micro-cracking and exfoliation of stones as well as erosion of building material surfaces (Sabbioni et al., 2010). The risk of thermoclastism is projected to increase in the Mediterranean region with climate change (Camuffo, 2019; Sabbioni et al., 2008; Sabbioni et al., 2010), notably for the widely used Carrara marble (Bonazza, Sabbioni, et al., 2009). Some adaptation has already been undertaken in the region, for example, the covering of archeological World Heritage Sites (WHS) in Malta by open-sided shelters (Figure 4). These prehistoric structures date to between the mid-4th and the mid-3rd millennium BC, using primarily Globigerina limestone, a relatively soft very porous local limestone (Bonazza, Sabbioni, et al., 2009; J. Cassar, 2016; Sabbioni et al., 2009). Exposure of these sites to solar radiation, and also rain, salts, and wind, since their excavations has led to their deterioration (Becherini et al., 2016). One of the mechanisms at play is here in fact thermoclastism (Brimblecombe, Bonazza, et al., 2011).
Change in Precipitation
Overview
Water is the most important decay factor for all buildings and especially the historic environment (Sabbioni et al., 2008). An increase in precipitation under climate change could lead to saturation of soils and overloading of gutters and downpipes, and hence a higher risk of damp penetration in historical materials, including masonry walls (Sabbioni et al., 2009). The penetration of water into porous materials can also result from condensation as well as from capillary action in the presence of soil moisture. Water ingress promotes material degradation through corrosion, biological activity, and subflorescence due to salt crystallization (Curtis, 2016; Sabbioni et al., 2008; Sabbioni et al., 2010).
Corrosion
More precipitation, particularly in a warmer climate, increases the corrosion of metals and vitreous materials and the surface recession of carbonate stones (Brimblecombe, Grossi, et al., 2011; Camuffo, 2019; Lefèvre, 2014; Sabbioni et al., 2010; Sabbioni et al., 2008), for example limestone and marble. Corrosion is a chemical phenomenon that causes gradual deterioration of materials by the action of water, usually together with salt (commonly chlorides) deposition, and is more prevalent in the presence of acid rain and higher atmospheric concentrations of carbon dioxide (CO2) for carbonate stones (Figure 4) (Sabbioni et al., 2010). The potential damage of corrosion to cultural heritage under climate change has been studied in Europe (Bonazza, Messina, et al., 2009; Brimblecombe & Grossi, 2009; de la Fuente et al., 2013; Grøntoft, 2011; Grøntoft & Cassar, 2020; Sabbioni et al., 2010; Tidblad, 2012, 2013) and Panama (Ciantelli et al., 2018).
Grøntoft (2011), predicted an increase in the corrosion of various metals, that is, steel, zinc, copper, and bronze, as well as Portland limestone and painted rendered surfaces for northern Europe, because of warmer and wetter conditions, but a decrease for the South of Europe where drier conditions are projected. Sabbioni et al. (2010) similarly predicted an increase in the corrosion of steel and bronze in urban areas for the North of Europe and a decrease for the South. They also found that the corrosion of zinc, copper, and lead is expected to be higher in areas with higher chloride deposition, such as in coastal areas and where de-icing salts are used during cold spells, while an overall decrease, although minor, in the corrosion of vitreous materials is projected for all of Europe. The former agrees with Tidblad (2012) and Tidblad (2013), who again projected a faster corrosion rate for carbon steel under climate change for the coastal areas of northern Europe, and a higher rate of zinc corrosion in the coastal areas of southern Europe.
For the façades of stone buildings in northern Europe, where an increase in precipitation is expected, there is a risk that this will cause even further corrosion of the surface of stone masonry buildings, as predicted for Portland limestone (Grøntoft, 2011), and low porosity carbonate stones (Bonazza, Messina, et al., 2009), such as marble and compact limestone (Sabbioni et al., 2010). ‘Clean’ rainfall has a pH of approximately 5.6 and is thus slightly acidic, causing degradation of carbonate stones, a phenomenon referred to as the karst effect (Baedecker et al., 1992). As the atmospheric concentration of CO2 increases due to human activities, Bonazza, Messina, et al. (2009) predicted an increase in karst recession of carbonate stones due to further acidification of rainfall. The damage function used in Bonazza, Messina, et al. (2009) was then applied by Ciantelli et al. (2018), who predicted that surface recession of a specific assemblage of carbonate stones utilized in the UNESCO WHS of Panamá Viejo and the Fortresses of Portobelo and San Lorenzo in Panama will increase.
Air pollution is often associated with surface soiling of the stone surfaces of historical buildings which is also corrosive (Grossi & Brimblecombe, 2007). Air pollution includes sulfur dioxide (SO2) and nitrogen oxides (NOx), which react with water to produce sulfuric and nitric acids (Haneef et al., 1992). Their presence can further decrease the pH of rainwater, causing acid rain (Sabbioni et al., 2006). Bonazza, Messina, et al. (2009) projected an increase in the recession of carbonate stone for most of Europe, but mainly through the karst effect, with the impact of air pollution considered to be less significant given that a decrease is foreseen for Europe, and mentioned that this recession will be more severe in mountainous regions because of greater precipitation. This agrees with Brimblecombe and Grossi (2009) who considered the effect of air pollution together with an increase in precipitation on the corrosion of limestone in London, and argued that because the air quality in London has improved in recent decades, there should be a shift in research emphasis from air pollution to climate change impacts. The better air quality is because of more stringent government regulations, resulting in a related decrease in SO2 emissions (Brimblecombe, Grossi, et al., 2011). M. Cassar and Pender (2005) also predicted increased corrosion of the façades of stone buildings for their two study regions in the UK based on a warmer and wetter climate only, while Grøntoft and Cassar (2020) predicted only a slight increase in the recession of Portland limestone theoretically exposed outdoors in the Mediterranean climate of Malta, and this in order to subsequently assess the recession of Maltese Globigerina limestone.
Biological Degradation
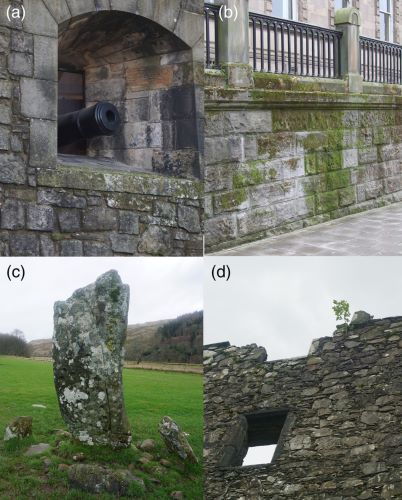
Changes in humidity influence the growth of microorganisms on stone (Figure 5) and wooden heritage materials (Sabbioni et al., 2009). Accordingly, a number of studies suggested that an increase in relative humidity in a warmer climate would aggravate biological degradation of cultural heritage (Ariño et al., 2010; Brimblecombe, Grossi, et al., 2011; Camuffo, 2019; Cutler et al., 2013; Gómez-Bolea et al., 2012; Grøntoft, 2019; Haugen & Mattsson, 2011; Lefèvre, 2014; Nijland et al., 2009; Prieto et al., 2020; Sabbioni et al., 2010; Smith et al., 2010; Viles, 2002; Viles & Cutler, 2012). Longer periods of wetness increase relative humidity and also moisture within materials, and together with rising temperatures create conditions favorable to various biological activity, causing deterioration of wooden historical buildings through biomass accumulation and decay by fungi, algae, mold, and lichens as well as by insects (Brimblecombe, Grossi, et al., 2011); termite attacks, can even lead to the collapse of timber structures (Sabbioni et al., 2008), and their geographical range is likely to expand further North in a warmer climate (Berenfeld, 2008).
In regions where a wetter climate is expected, such as the boreal and temperate regions, an increase in biological growth on heritage assets is again projected (Viles & Cutler, 2012). Accordingly, Ariño et al. (2010), Sabbioni et al. (2010) and Gómez-Bolea et al. (2012) predicted an increase in biomass accumulation on hard acid stones in the boreal region (i.e., northern Russia, Scandinavia, and Scotland) due to warmer and wetter conditions. Likewise, in the tropics, Ciantelli et al. (2018) projected a slight increase in biomass accumulation also on similar stones on the northern shore of Panama. In regions where the climate will likely become drier, such as the Mediterranean region, but also the Middle East, the Caribbean, and southern Africa, a reduction in the risk of biological degradation is expected, as environmental conditions influence the composition and activity of biotic communities, with the type of substrate playing a secondary role (Viles & Cutler, 2012).
The UK is located in a temperate region where a wetter climate is projected, particularly in the winter. Because an increase in precipitation will lead to longer periods of wetness of material surfaces, Smith et al. (2010) projected an increase in algal biofilms on stone buildings, as wetter conditions increase the risk of moisture penetration into porous stones, favoring algal growth; a result in agreement with McCabe et al. (2013). However, Cutler et al. (2013) warned that the relationship between moisture level and the greening of stones is more complicated, in fact, they did not find any evidence that the growth of algal biofilms causes weathering of sandstone buildings in Belfast, even suggesting that the green biofilms could protect the stones. In Galicia, Spain, which similar to the UK experiences a temperate oceanic climate, one would expect no change in the amount of biomass on granite in the future, because of projections toward a drier climate; however, on the basis of an experimental study, it was concluded that the biofilm composition might change and result in faster stone degradation (Prieto et al., 2020). For Northern Ireland, Adamson et al. (2010) found that sandstone buildings in regions with higher precipitation and hence higher moisture levels were more affected by biological soiling and stone decay.
Fungal attacks are one of the main causes of wood deterioration (Sabbioni et al., 2010). Haugen and Mattsson (2011) identified temperature, air humidity and the moisture content of wood as the three main variables leading to bio-deterioration due to fungi, mold and insects, with the moisture content of wood being affected by increased precipitation, wind-driven rain (WDR) and floods. WDR is the component of rain that falls obliquely onto surfaces (Orr et al., 2018). Sabbioni et al. (2010) also indicated that wooden buildings in the North and East of Europe as well as the Northwest of the British Isles would be at greater risk of fungal attacks as temperature and humidity increase. In Norway, Grøntoft (2019) predicted an increase in rot risk for wooden historical buildings. In contrast, a lower risk for fungal growth for the South and West of Europe is projected, due to the drier conditions that are expected for this region (Sabbioni et al., 2010).
Changes in temperature and rainfall alter the distribution and abundance of lichens (Giordani & Incerti, 2007), and the lichen species richness (Ariño et al., 2010). Lichens can grow on stone substrates, as illustrated in Figure 5. The development of lichens’ thalli (i.e., the vegetative tissue of the lichen) cause solubilization of certain minerals, which results in the loss of a few millimeters of mineral substrate and de-cohesion of mineral grains (Sabbioni et al., 2010). Ariño et al. (2010) and Sabbioni et al. (2010) projected a decrease in lichen species richness in Europe under climate change with an exception for the northern regions where an increase is expected. This is because warmer temperatures and exposure to the sun, as well as low relative humidity conditions can cause desiccation of the lichen’s thalli.
Salt Crystallization Cycles
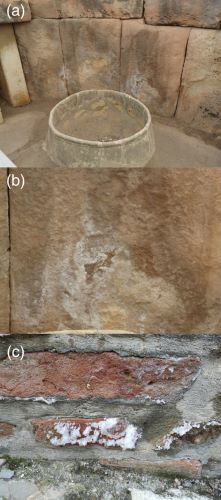
Soluble salts can dissolve and recrystallize with changes in temperature and humidity, creating salt crystallization cycles. Some salts can change their structure during their hydration and dehydration cycle, for instance, sodium sulfate (thenardite) and sodium sulfate decahydrate (mirabilite) (Rodriguez-Navarro et al., 2000). The mirabilite phase can exert very high crystallization pressures on pores in a stone (Espinosa et al., 2008), such repeated cycles can cause stress and, in the long run, decay. Fluctuating changes in precipitation and temperature, in the presence of soluble salts in stone materials, will lead to an increase in the number of salt crystallization cycles and hence more damage. The salt load in a building material or structure can also increase by greater precipitation leading to saturation of soils coupled with capillary action (Haugen & Mattsson, 2011; Figure 6).
Even small variations in relative humidity can have a significant influence on the number of damaging salt crystallization cycles. When the relative humidity drops below the deliquescence point of certain soluble salts, these change from a solution to a crystalline phase. The mechanism of damage is that when salts crystallize, the change in volume can exert mechanical stress on the materials (i.e., subflorescence) or compromise the readability of artifacts if salts crystallize on surfaces (i.e., efflorescence) (Brimblecombe, 2013; Brimblecombe, Grossi, et al., 2011; Sabbioni et al., 2010; Sabbioni et al., 2008; Viles, 2002). Brimblecombe and Grossi (2007), Sabbioni et al. (2010), and Camuffo (2019) predicted an increase in soluble salt crystallization events for almost all of Europe, but especially Central Europe, because of the projected decrease in relative humidity during the summer. The impact of weathering due to changes in the number of salt crystallization cycles, under climate change, was also specifically studied at heritage sites in France (Menéndez, 2018), The Netherlands (Nijland et al., 2009), and Panama (Ciantelli et al., 2018), where areas were identified where changes in salt crystallization cycles would subject materials to greater stress.
Change in Wind and WDR
Changes in wind speed and direction, as well as wind gusts during storms can cause enormous damage to heritage buildings and archeological sites, as they can impact the structures and potentially cause their collapse (Blankholm, 2009; Brimblecombe, Bonazza, et al., 2011; Grossi et al., 2011; Ravankhah et al., 2019; Sabbioni et al., 2008; UNESCO World Heritage Centre, 2007). Wind can transport pollutants, salts and sand, which damage heritage materials (Sabbioni et al., 2010), with the risk of eolian sand erosion prevailing in arid environments (Qu et al., 2014). Wind and WDR can cause abrasion of building materials and archeological rock art (ICOMOS Climate Change and Heritage Working Group, 2019; Sabbioni et al., 2010) in other environments, also through erosion by wind carried particles (M. Cassar & Pender, 2005; Erkal et al., 2012; Haugen & Mattsson, 2011). WDR also increases the risk of water penetration into porous materials, which can influence the migration of salts and cause discolouration of the walls of historical buildings, as noted for Norway, due to efflorescence resulting from salt crystallization (Haugen & Mattsson, 2011). Other similar studies across Europe were by Sabbioni et al. (2010) and Brimblecombe, Grossi, et al. (2011), and Erkal et al. (2012). Regional studies in the UK showed that the occurrence of WDR is not expected to change but it is predicted to become more intense, leading to a greater likelihood of moisture ingress into buildings, also from the over-spilling of gutters (Orr et al., 2018). Such increase in the intensity of precipitation was also considered by Parkin et al. (2015) in Scotland, who suggested that this, coupled with the action of wind and ice, would further enhance the erosion of earth-built heritage buildings. In Romania, Mosoarca et al. (2017) predicted damage to historic assets due to WDR, particularly when coupled with hailstorms, while Nik et al. (2015) predicted that WDR would lead to more moisture accumulating in the walls of buildings in Sweden. Beyond Europe, Pearson (2008) predicted increased damage to timber and iron vernacular historical buildings due to WDR in Australia.
Four Impacts of Gradual Changes in Climate on Indoor Cultural Heritage and Collections
Overview
Climate change is a problem also for building interiors (Nik et al., 2015), as external climatic conditions can directly influence internal conditions in uncontrolled buildings, which many historic buildings are. Changes in internal thermo-hygrometric conditions will cause variations in the mechanical, chemical and biological decay of the interiors and the objects and collections they house. Museums host a variety of collections, both organic and inorganic, often located in historical buildings lacking modern climate control measures; therefore the potential climate change impacts on the internal conditions also need to be assessed (Bertolin & Camuffo, 2015; Huijbregts et al., 2011; Tornari et al., 2013). These collections include paper-based collections, including archives (Turhan et al., 2019), and can also be exposed to flooding (Mazurczyk et al., 2018; Tansey, 2015). The potential impacts of climate change on museum collections was the focus of roundtable discussions at the 2008 congress of the International Institute for Conservation of Historic and Artistic Works (IIC). D. Saunders (2008) summarized the outcomes of the deliberations, highlighting that previous research on climate change impacts had focused on outdoor cultural heritage, and stressed the paucity of research on the indoor heritage, notably museum collections. This topic has since then gained the attention of many (Ashley-Smith, 2013; Bertolin & Camuffo, 2014, 2015; Huijbregts et al., 2013; Huijbregts et al., 2014; Lankester & Brimblecombe, 2012a; Leissner et al., 2014; Leissner et al., 2015; Loli & Bertolin, 2018; Melin et al., 2018; Sesana et al., 2019), with all these studies focusing on Europe where an increase in average indoor temperature is generally foreseen, together with small changes in indoor relative humidity, notably a decrease in the South and an increase in Eastern and coastal regions (Bertolin & Camuffo, 2014; Huijbregts et al., 2013; Leissner et al., 2015). These changes have the potential to lead to a gradual and continuous deterioration of the artifacts housed in historical buildings (Huijbregts et al., 2013; Lankester & Brimblecombe, 2012a) through an intensification of deterioration mechanisms.
Mechanical Degradation
Freeze-Thaw Cycles
Freeze–thaw cycles can occur in uncontrolled indoor environments, albeit rarely, causing mechanical degradation of stone, mortar and ceramic artifacts housed there, and also including those comprising the built envelope of masonry buildings. Previous studies on this topic related to climate change are limited to Europe and have found that the influence of freeze–thaw cycles on indoor environments will decrease in Central and Eastern Europe, but with no change projected for the Atlantic region of Western Europe (Ashley-Smith, 2013; Bertolin & Camuffo, 2015; Loli & Bertolin, 2018; Sesana et al., 2019).
Salt Crystallization Cycles
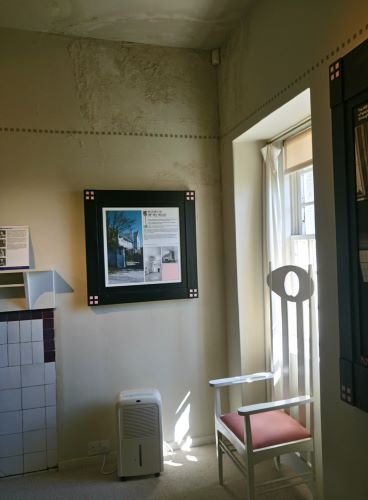
The mechanisms of damage inside and on the outside of buildings, are similar, occurring when the indoor environment reaches a level of relative humidity favorable for salt crystallization. In the presence of specific soluble salts, efflorescence, and subflorescence can occur, for example, on surfaces and decorations of the internal walls of historical buildings subject to damp penetration (Huijbregts et al., 2013; Figure 7). Fluctuations in relative humidity and a change in its range of variability will affect the occurrence of hydration-dehydration phase changes in certain soluble salts (e.g., damaging thenardite-mirabilite cycling; Ashley-Smith, 2013; Bertolin & Camuffo, 2015). There is a considerable geographic variability in projections of change, depending on local climate. Leissner et al. (2015) projected that this phenomenon will increase in Central Europe and in other areas of Northern Europe, but decrease in the Alps. Lankester and Brimblecombe (2012a) projected variable changes in the occurrence of salt crystallization cycles depending on location, including an increase in northern Spain and Prague, but a decrease for sites in Britain. The frequency of thenardite/mirabilite cycles is also likely to increase in the Northeast of Europe (Bertolin & Camuffo, 2014).
Mechanical Damage on Wooden Materials and Historic Masonry Buildings
Climate change induced variations of indoor temperature and relative humidity in historical buildings can also lead to physical damage of hygroscopic wooden materials (Bertolin et al., 2015; Bratasz et al., 2012; Huijbregts et al., 2013; Melin et al., 2018), and to historic masonry buildings (Cavalagli et al., 2019). When relative humidity is high, wood tends to absorb moisture and mechanical stress occurs during cycles of swelling and shrinkage. This can also affect associated materials, such as coatings on wooden panels or furniture, which can lead to detachment from substrates and damage to painted surfaces (Ashley-Smith, 2013; Bertolin & Camuffo, 2014) or gilded parts. Accordingly, Bertolin and Camuffo (2014) projected an increase in mechanical damage for hygroscopic materials with a higher risk in the summer season for Southern Europe due to increased fluctuations in humidity, while Huijbregts et al. (2013) and Huijbregts, Schellen, van Schijndel et al. (2015) predicted an increase in the detachment of paint on wooden art objects in historical buildings across Europe. Similarly, Rajčić et al. (2017) and Muñoz González et al. (2020) anticipated an increase in mechanical damage to pictorial layers and wooden sculptures and altarpieces, exemplified by their study of the St. Barbara Chapel in Croatia and St. Francis of Assisi in Italy. Cavalagli et al. (2019) further predicted worsening of structural damage to Consoli Palace, an historic masonry building, because of an increase in indoor temperature and moisture conditions.
Chemical Degradation
Where conditions become warmer and more humid, as predicted by climate change scenarios, rates of chemical reactions can increase. This can affect heritage materials made of paper and silk, as well as colored photographs. In the presence of moisture, and particularly in a warmer climate, acidic paper or paper affected by air pollution can undergo the breakdown of its the cellulose fibers, resulting in paper degradation in books and manuscripts. Lankester and Brimblecombe (2012a) projected an increase in this deterioration in different European cities due to climate change; this was also the case for Turhan et al. (2019) studying the collections of a library in Izmir, Turkey. Bertolin and Camuffo (2014) also predicted a decrease in the lifetime of paper, particularly for Northern Europe and the Alps, as well as an increase in the risk of silk damage along the Atlantic coastline of Europe. Many heritage objects such as wall decorations, furniture fabrics and costumes are made of silk, with their lifetime shown by Ashley-Smith (2013) to be dependent on relative humidity and temperature. Warmer and more humid internal conditions also alter the color of photographs, particularly in the presence of acidic pollutants, with Ashley-Smith (2013) and Bertolin and Camuffo (2014) projecting an increase in discolouration for the South of Europe. Furthermore, Coelho et al. (2019) projected an increase in chemical degradation of artifacts (in the broad sense) in Seville (Spain) and Oslo (Norway).
Biological Degradation
A growing risk for the indoor environment in a changing climate is degradation by mold and insects (Ashley-Smith, 2013). Various authors (Bertolin & Camuffo, 2014; Huijbregts et al., 2012; Huijbregts et al., 2013; Huijbregts, Schellen, Martens, et al., 2015; Lankester & Brimblecombe, 2012b; Leissner et al., 2015) project an increase in mold growth on the interiors and collections of historical buildings in the winter for the North of Europe, because of an increase in relative humidity, but a decrease in the summer, with Leissner et al. (2015) indicating that the British Isles and Scandinavia will be the most affected in this manner. The risk of mold decay is expected to remain low in Southern Europe because of the projected warmer and drier climate (Huijbregts et al., 2013). Bertolin and Camuffo (2014), in addition, projected an increase in insect activity for all of Europe as the climate warms, but an increment in decay activity due to insects in regions where humidity levels are predicted to increase such as Northern Europe. Accordingly, Stengaard Hansen et al. (2012) expected a rise in biodegradation of organic objects in museums in Scandinavia because of the brown carpet beetle Attagenus smirnovi as the climate warms.
Impact from Changes in the Natural Physical Environment on Cultural Heritage
Overview
Catastrophic changes in the natural physical environment resulting from climate change, such as flooding, rising sea level and its associated coastal impacts, and landslides can have great impacts on cultural heritage, both outdoor and indoor.
Flooding
Flooding is one of the most important consequences of climate change for cultural heritage in Europe (Historic England, 2015). The need for reducing and managing the risks of flooding on cultural heritage was thus included in Directive 2007/60/EC of the European Parliament and of the Council of 23 October 2007 on the assessment and management of flood risks.3 The frequency and magnitude of floods are projected to increase in Europe and Sabbioni et al. (2010) defined particularly prone regions. Floods amplify other losses in coastal areas, affecting different heritage typologies (Blankholm, 2009; M. Cassar & Pender, 2005; Dassanayake et al., 2018; Glaser et al., 2010; Haugen & Mattsson, 2011; Kincey et al., 2008; Ravankhah et al., 2019; Snow, 2014; Wang, 2015). They drastically increase moisture in historical materials and structures, causing physical, chemical and biological degradation (Dassanayake et al., 2018). Changes in soil moisture can accelerate deterioration processes, for example, the growth of fungi affecting wooden heritage and the mobilization and crystallization of salts leading to degradation of stone surfaces (Haugen & Mattsson, 2011; Sabbioni et al., 2008).
Penetrating moisture in buildings during a flood for a prolonged period of time, can potentially raise the humidity in the building’s interior and additionally cause degradation of its collections (Huijbregts et al., 2014); this can be exacerbated by inefficient drainage systems, originally designed for less water and overflow. Floods can also produce or worsen erosion, further aggravating the decay of buildings. Overdevelopment, and using impermeable ground surfacing in historical cities, can compromise an urban drainage system by preventing infiltration of rainwater to the deeper substrata and thus causing damage due to increasing runoff. Kaspersen and Halsnæs (2017) have foreseen an intensification of urban flooding in the city of Odens in Denmark and indicated that cultural heritage would be a key sector affected. The effect of changing fluvial behavior on the other hand has been predicted to increase impacts on river flood plain archeological sites (Howard et al., 2008). There is evidence that past climate variations resulted in changes in catchment area erosion and downstream deposition that can threaten (by erosion) but also protect archeological sites (through burial). High groundwater levels are also known to favor better preservation of buried archaeology (Howard et al., 2008), hence fluctuations of groundwater related to climate change affect river discharge, in rural, and also in urban areas with their modified runoff characteristics, and have the potential to stress archeological resources.
Balica et al. (2012) projected that the number of cultural heritage sites exposed to flooding will increase globally. Ghozali et al. (2016) mentioned flooding as an important climate change risk for the UNESCO WHS of Ayutthaya in Thailand and the historical city of Samarinda in Indonesia. In Laos, Fumagalli (2020) recently highlighted the potential risk of changes in flooding due to increased precipitation and an increase in the frequency and intensity of cyclones to the UNESCO WHS of Luang Prabang. For WHS in the UK Howard (2013) and Howard et al. (2016) highlighted areas where adaptation against the impacts of climate change will be required in the Derwent Valley Mills area in Central England. Tansey (2015) and Mazurczyk et al. (2018) anticipated an increase in the impacts of flooding on archives in the United States. Flooding can also be caused by melting glaciers; Berenfeld (2008) predicted that in the Himalayas this will affect traditional wood and mud temples. Furthermore, the mud architecture of Yemen is expected to be at risk of flooding under changing climatic conditions (Al-Masawa et al., 2018), as well as rock art sites in Australia (Rowland, 1999).
Slope Instability and Landslides
More extreme rainfall under climate change will increase the risk of gully erosion (Nicu, 2016, 2017) and landslides (Cigna et al., 2016; Crozier, 2010; Gariano & Guzzetti, 2016; ICOMOS Climate Change and Heritage Working Group, 2019). For this reason, the risk of climate change to ground stability causing landslides, and their consequences on cultural heritage, have been referred to in the literature (Cigna et al., 2016; Ravankhah et al., 2019; Sabbioni et al., 2010; UNESCO World Heritage Centre, 2007). Sabbioni et al. (2010) defined areas subject to this risk and projected an increase across Europe. At the regional or local scale, Cigna et al. (2016) studied the Derwent Valley Mills in the UK under climate change and projected an increase in landslide risk, as a consequence of extreme rainfall predicted for the UK, agreeing with the earlier analysis of Sabbioni et al. (2010) at the continental scale. Ravankhah et al. (2019) included landslides in a number of identified geological hazards, that may influence a site on the Greek Island of Crete in a changing climate. Furthermore, many heritage rupestrian sites, such as the WHS of Petra in Jordan, are carved in cliffs and are inevitably exposed to slope instability and rockfall, in addition to the ubiquitous weathering of the rocks (Margottini et al., 2016); nonetheless, potential future changes in rockfall hazards due to climate change have yet to be investigated in this case.
SLR and Associated Coastal Impacts
Sea Level Rise
Rising sea level poses a significant threat to cultural heritage (Perez-Alvaro, 2016) situated in coastal areas, and Marzeion and Levermann (2014) have estimated that 19% of UNESCO WHS globally could be lost to inundation in a 3°C warming scenario. Studies in Europe on this topic have to date focused on the Mediterranean region. Reimann et al. (2018) investigated the potential risk of flooding due to SLR for 49 WHS in this region and Ravanelli et al. (2019) focused on the archeological site of Motya in Sicily. Investigating similar scenarios in other areas, García Sánchez et al. (2020) mentioned that SLR is threatening coastal fortifications in the Canary Islands, and Heilen et al. (2018) investigated the impacts of SLR on archeological sites in Germany. In the Americas, Anderson et al. (2017) identified the number of archeological sites at risk of being submerged in the Southeast of the United States; the risk of SLR on archeological sites in Trinity Bay, Texas, was studied by Elliott and Williams (2019) and in Puerto Rico Ezcurra and Rivera-Collazo (2018) investigated such effects on all cultural heritage typologies. On the Atlantic coast of Georgia, Heilen et al. (2018) projected an increase in the number of archeological sites which can be impacted by SLR. On the other side of the world, in Bangladesh, Berenfeld (2008) predicted an increase in the risk of flooding on historical buildings due to loss of natural barriers such as mangrove forests coupled with rising seas, while in Australia, Rowland (1999) highlighted the risk that an increase in sea level would cause to aboriginal sites, low-lying historical buildings and shipwrecks.
Cultural heritage assets situated underwater, including sunken coastal cities and settlements, shipwrecks and archeological materials, is also at risk from SLR (UNESCO, 2011), through shallow-water erosion and a retreat of benthic species. Such as seagrass, which acts as an anchor for submerged sites, further increasing erosion and affecting the stability of the underwater heritage (Garner et al., 2015; M. I. Saunders et al., 2013; Wright, 2016). SLR will also increase the frequency of extreme events, such as storm surges (Hunter, 2009) resulting in higher waves, and affecting the sediment balance in coastal regions and the stability of underwater archeological sites and monuments (Dunkley, 2013a; Wright, 2016).
Coastal Erosion and Coastal Flooding
Rising sea level together with a higher frequency of storm surges will increase coastal erosion, causing re-shaping of the coast, and a further increase in the frequency of coastal flooding, impacting coastal heritage sites (M. Cassar & Pender, 2005; Dassanayake et al., 2018; Dawson et al., 2017; Graham, Hambly, et al. (2017a, 2017b); Harkin et al., 2020; Murphy et al., 2009; Pollard-Belsheim et al., 2014; Ravankhah et al., 2019; Westley et al., 2011). Climate change can also worsen the effects of eolian and marine processes, such as wave action and currents, on shoreline and submerged archeological sites, increasing erosion and potentially leading to the collapse of heritage structures (Mattei et al., 2019). Associated re-shaping of the shore can influence different heritage typologies, some of which may disappear due to erosion and coastal retreat, but also due to sediment accumulation (Daire et al., 2012; Dassanayake et al., 2018; Pearson, 2008; Reeder-Myers, 2015; Sabbioni et al., 2008). Limited flood defenses and their poor maintenance can also lead to erosion, not only in coastal areas, but also on riverbanks (M. Cassar, 2005; M. Cassar & Pender, 2005; Haugen & Mattsson, 2011; Vojinovic et al., 2015), with the potential loss of heritage assets. Storms can breach or even lead to the collapse of protective seawalls and jetties, with the velocity of the resulting flood waters potentially causing collapse of heritage sites (Westley et al., 2011).
Some of the archeological sites situated along the Atlantic coastline of Europe could be lost in the next few years because of an increase in storminess, SLR and coastal erosion, notably the Iron Age remains in Shetland, Orkney, and the Hebrides in Scotland (Daire et al. (2012). In the latter country alone it is estimated that there are 12,000 archeological sites at risk from coastal erosion (Westley et al., 2011). Daire et al. (2012) also developed a methodology to assess the vulnerability of archeological sites, taking into account various factors, such as distance from the cliff and exposure of the site to wave action, which Shi et al. (2012) also applied to the Vilaine Estuary in Brittany, France. Work has also been undertaken in other areas to identify archeological sites at risk of SLR and associated coastal impacts, notably in the UK (Cook et al., 2019; Westley & McNeary, 2015), Italy (Anzidei et al., 2020), Newfoundland and Labrador (Pollard-Belsheim et al., 2014; Westley et al., 2011), California (Reeder et al., 2010; Reeder-Myers, 2015), Australia, and New Zealand (Bickler et al., 2013; Carmichael et al., 2017; Rowland, 1992).
Salt damage in addition threatens cultural heritage assets located in proximity of the coast (Howard et al., 2008). Venice is the emblematical example of a city whose foundations are directly in contact with seawater. This leads to the migration of salts into the masonry walls and hence the risk of degradation through salt crystallization (Borg et al., 2014; Camuffo et al., 2014; Di Sipio & Zezza, 2011). In a city such as Venice with many historical buildings with wooden elements, including foundation piles, SLR can also cause an increase in biological degradation by marine woodborers (Tagliapietra et al., 2019).
Changes in Ocean Properties: Temperature, Salinity, and Acidity
Warmer Sea Surface Temperatures (SSTs) could cause changes in water currents, oxygen levels, and salinity (Perez-Alvaro, 2016; Wright, 2016). In addition, the absorption of atmospheric CO2 by the oceans leads to its acidification and could affect underwater heritage, notably shipwrecks and submerged archeological sites. Ocean acidification influences the iron and steel of shipwrecks by increasing the rate of corrosion (Dunkley, 2013a, 2015; Perez-Alvaro, 2016). A change in salinity expected under climate change as a result of an intensification of the hydrological cycle enhancing precipitation and evaporation (Durack et al., 2012; Rhein et al., 2013; Spalding, 2011), together with warmer SSTs could cause an increase in the presence of organisms such as shipworms, causing damage to underwater wooden structures (ICOMOS Climate Change and Heritage Working Group, 2019). In recent decades, there has been an increase in the occurrence of one species, the Teredo navalis in the Baltic Sea (Appelqvist et al., 2015), where thousands of shipwrecks of archeological importance have been preserved, with climate change suggested as a cause for this increase (Björdal, 2012; Björdal et al., 2013). Similarly, the presence of another species of shipworm, Lirodus pedicellatus, was identified on wrecks along the South coast of England, believed to have migrated from lower latitudes as a result of increasing temperatures (Harkin et al., 2020). Combined changes in the acidity, temperature, and salinity of the oceans, together with changes in nutrient availability could also impact biological mechanisms causing wood decay by fungi, but there is a lack of research into how underwater heritage might be affected by this risk in the future (Björdal, 2012; Dunkley, 2013a, 2013b).
Permafrost Thawing
In a warmer climate, cultural assets that are anchored in permanently frozen ground will be subjected to annual freeze–thaw cycles and ensuing degradation (Blankholm, 2009; Grossi et al., 2007). Archeological heritage, including burial sites located in polar environments, at high latitudes and in mountainous areas are also expected to be affected by microbial decay under climate change (ICOMOS Climate Change and Heritage Working Group, 2019). Another threat is the heat produced by microbes, which accelerates in warmer soils (Hollesen et al., 2018), as well as the softening and possible subsidence of buildings foundations (ICOMOS Climate Change and Heritage Working Group, 2019). Permafrost thawing also exposes the frozen archeological assets to erosion (ICOMOS Climate Change and Heritage Working Group, 2019), and also increases the susceptibility of coastal areas to such damage (Wobus et al., 2011), thereby affecting more archeological sites located on the coast (Hollesen et al., 2018; Hollesen, Matthiesen, & Elberling, 2017; Hollesen, Matthiesen, Madsen, et al., 2017; Lantuit et al., 2012). This is in addition to SLR, together with the extension of the ice-free season, which can lead to higher and stronger waves, and an increase in coastal erosion (Barr, 2008; Lantuit & Pollard, 2008). Thus, the risk of coastal erosion is becoming a serious threat to cultural heritage preservation in the Arctic (Barr, 2008; Nicu et al., 2020; Sesana et al., 2018), where there are approximately 180,000 registered archeological sites (Hollesen et al., 2018) and probably many more unknown ones. The loss or damage of archeological sites has already been reported across the region (Berenfeld, 2008; Flyen, 2009; Friesen, 2015; Grossi et al., 2007; Jensen, 2017; Jones et al., 2008; O’Rourke, 2017; Sabbioni et al., 2010).
Droughts and Extreme Heat
Droughts together with extreme heat can increase the risk of ignition of fires and their propagation (Sabbioni et al., 2008, 2010). In Europe, Sabbioni et al. (2010) predicted an increase in cultural heritage exposure to fire for the Iberian Peninsula. According to the European Forest Fire Information System, the climate across almost all of Europe is projected to change toward conditions more favorable toward the occurrence of fire. This growing threat to cultural heritage was emphasized during a seminar organized on June 26–28,2019 by the International Centre for the Study of the Preservation and Restoration of Cultural Property (see ICCROM),4 as well as in an ICOMOS publication focusing on the impact of fire on cultural heritage in a national park in Australia (Pearson, 2015). The author of the latter publication had earlier highlighted the risk that increased bushfire frequency can have on cultural heritage, also for Australia (Pearson, 2008). Fire causes material loss and deformation of cultural heritage assets, and may also increase the probability of cracking or splitting in built structures (ICOMOS Climate Change and Heritage Working Group, 2019). Under extreme heat, stones can face both macro (e.g., cracking of stones, soot accumulation, color change in stone containing iron) and micro (e.g., mineralogical and textural changes) degradation processes, leading to potential structural instability. The long-term impacts include weakened stones and increased susceptibility to other deterioration processes such as salt weathering and through temperature cycling (Gomez-Heras et al., 2009). Furthermore, drought conditions will lead to desiccation of soils and unfired building materials as well as structural implications for the foundations of historical buildings and archeological sites (Brimblecombe, Grossi, et al., 2011). Berenfeld (2008) predicted an increase in drought and desertification under climate change in Africa, leading to an increase in the risk of encroaching sands that cover and erode heritage materials, and an increase in the risk of flash flooding. Pearson (2008) highlighted the need for research in arid places such as Australia given the associated risk of desertification caused by an increase in drought frequency and soil desiccation coupled with increased wind speed and ensuing erosion.
Discussion and Conclusion
Climate change can exacerbate exposure of cultural heritage to climatic stressors due to gradual changes in climate, SLR, and the occurrence of extreme events. In reports published over a decade ago, UNESCO mentioned the need for more research on such impacts (UNESCO World Heritage Centre, 2007, 2008), and, accordingly, an increase in the number of published articles on this topic followed. This review is global, albeit it is dominated by European research given that this has been the geographical focus of most research accomplished to date. Nonetheless, our search has identified a number of studies focusing on North America, Australia, and New Zealand, and clearly revealed a paucity of studies originating from other regions. This is a notable limitation of this review, but reflects the global state of research into the impacts of climate change on cultural heritage. This study surveys the climate change impacts on the tangible cultural heritage, as investigated in the scientific literature. It does not consider uncertainties in climate change projections, nor the vulnerability of cultural heritage sites to such changes. This review found that most studies were limited to the use of one climate change scenario and one climate model without considering the potential uncertainties in how the climate might evolve. For parts of Europe, for instance, there is disagreement amongst models not only in the magnitude of change in future precipitation, but also in the direction of change. For this reason, one should normally use an ensemble of models to capture uncertainties. Nonetheless, this study can be a useful tool to inform risk and vulnerability assessments when other factors such as visitor pressure and maintenance are also included (e.g., Sesana et al. (2020)).
The review first examines how gradual changes in climatic variables such as temperature, precipitation, relative humidity, and wind are projected to affect degradation mechanisms on heritage assets exposed to the outdoor environment. These impacts can be summarized as follows:
- Water is the main agent of material degradation, at least in Europe. An increase in precipitation and other water related variables such as humidity cycles and periods of wetness, when coupled with warmer temperatures, can cause or enhance different decay mechanisms, for instance, corrosion, biological degradation, warping and splitting of hygroscopic materials, and efflorescence and subflorescence due to salt crystallization.
- An increase in wind and WDR will also affect cultural heritage, particularly in the presence of sand, salt, and atmospheric pollutants. This can lead to surface abrasion, increased water penetration, structural damage, and potentially the collapse of structures.
- A warmer temperature may result in an increase in the number of freeze–thaw cycles, and/or an increase in the diurnal temperature ranges affecting the occurrence of thermoclastism. This may intensify physical weathering of stone and ceramic materials.
The indoor environmental conditions of nonclimatised historical buildings will also be changed. The impacts of altered interior heritage environments due to climate change were considered as follows:
- Different temperature and relative humidity cycles can influence the mechanical, chemical and biological decay of interior heritage assets. Examples include changed freeze–thaw and salt crystallization cycles that can affect building envelopes, but also their collections. The latter can also be exposed to water-related risks, notably as a result of flooding.
- For the same reasons, the chemical degradation of organic heritage assets such as those made of silk and paper, as well as color photographs, might increase in certain areas.
- An increase in indoor temperature and humidity will increase biological degradation as it can create conditions favorable to mold growth and insect activity.
- The corrosion of metals and the degradation of glass can also be affected.
In addition, climate change may alter the natural physical environment with serious consequences for the preservation of cultural resources:
- Gradual changes, such as ocean warming, SLR and its associated coastal impacts, and the thawing of permafrost, can lead to episodic major impacts. These include changing occurrence of floods, droughts, heat waves, and landslides with direct, sometimes catastrophic, impacts on heritage assets. Indirect effects, such as increase in salt crystallization episodes and corrosion of metals, can also occur.
- Desertification is also expected to increase in a warmer and drier climate, with its associated risk for cultural heritage.
- Some risks in this category are yet under-researched and have not been addressed in the literature cited as part of this review.
Furthermore, there are synergisms that occur between various parameters, leading to direct and indirect, immediate and long-term, effects on a wide variety of materials, objects, buildings, and sites.In conclusion, this review can be used to inform risk and vulnerability assessments of cultural heritage sites to climate change, which are now becoming more pressing in view of the inevitable task of adaptation, particularly in the light of the recent ICOMOS’ climate and ecological emergency declaration calling for urgent action to protect cultural heritage from the impacts of climate change. Further research is recommended in regions with a paucity of research, for example, Asia, Africa, and Central and South America, and in estimating uncertainties in climate change impacts on cultural heritage at the local scale to provide examples of potential uncertainties and reliability, as well as on providing best practice information and tools to manage cultural heritage in a changing climate.
See endnotes and bibliography at source.
Originally published by WIREs Climate Change 12:4 (July/August 2021) under the terms of a Creative Commons Attribution 4.0 International license.